Current Investigations In Cancer Cell Biology Part IV: Why Is Fighting Cancer So Hard?
Facing a Mutant Mirror
Preface
This post is part of a series of essays I have written in MCB3842W: Current Investigations In Cancer Biology this semester. If you find yourself lost in any of the terminology or interested in the background information, check out my previous posts on Relative Risk vs. Absolute Risk, Genetics 101, and Mutations 101. Also, stay tuned for my final essay for this class where I will sum everything up by describing the Hallmarks of Cancer.
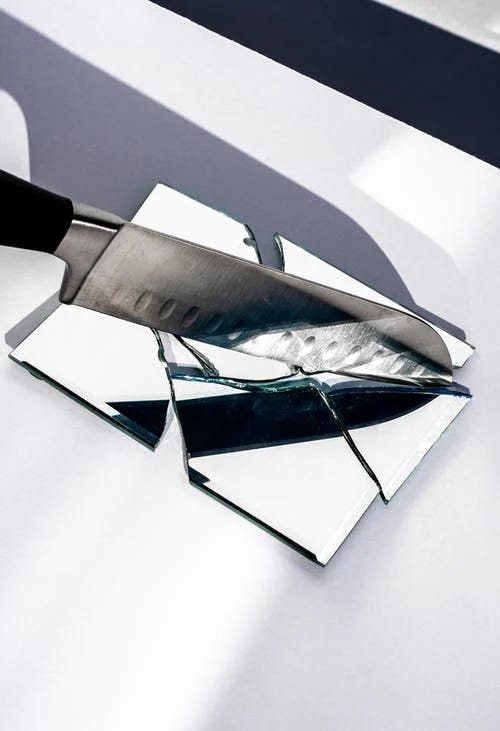
Allow me to take you back a couple hundred thousand years: the hot African sun relentlessly shines down on your skin, your hungry stomach growls for food, and a pack of eager hyenas stares you and your family down as if you are a pu pu platter; furthermore, and most importantly, there is no bottled Poland Spring, Stop&Shop, or law enforcement to rescue you from any of these problems. We weren’t the biggest, the strongest, nor the fastest, but in the end, humans came out on top in these environments because we were the most adaptable. We evolved to our surroundings, developing cognitive abilities that led to valuable inventions and innovations, like fire, tools, and social cooperation and structure. For this reason, we found ourselves on the top of the food chain — we became dangerous and widely feared predators. Now, 200,000 years later, it is not lions, tigers, and bears that we fear in the Developed World, nor is it the threats of bacterial infection, starvation, or the raging elements of nature; instead, our biggest opponents come in the forms of chronic conditions we develop over our lifetimes, such as neurodegenerative disease, metabolic dysfunction, and cancer. Interestingly, many of these diseases — such as type 2 diabetes, cardiovascular disease, and obesity — result from surpluses in the essential resources that we once fought to the bone for in our evolutionary battle for survival all those years ago. The case of cancer is especially interesting, in that cancer cells reflect the tenacious resilience that pushed us through those hot and hungry days in the wilderness: they are tenaciously adaptive, unremittingly resilient, and unapologetically innovative.
If you have been following along in this Cancer 101 series, then you now understand the basic genetic concepts that underlie cancer; however, you may feel like we have not made any headway on the question we asked in the first place: what is cancer? The simple textbook response to this question is that cancer is a disease of overgrowth, in which genetic mutation drives rogue cells to uncontrollably multiple and disturb proper physiology. The more nuanced and varsity-level response is that cancer is not a disease at all; rather, it is a category of diseases, each of which varies from system to system, from patient to patient, and even from tumor to tumor within the same patient. This week, we will move from the fundamentals of genetics and mutations to the frontier of cancer research, where researchers are recognizing how diverse and adaptable cancers can be.
This past September, researchers out of the Garvan Institute of Medical Research published a study in the journal Nature Genetics describing their single-cell RNA sequencing of samples from 26 different breast cancer tumors, as well as how their results impact their field’s perception of cancer as a whole. Single-cell RNA sequencing, or scRNA-seq, is a type of genetic screening, in which scientists can separate a piece of tissue into single cells, record those cells’ genetic characteristics, and use that data to draw conclusions about the cells themselves, as well as the original piece of tissue. In regards to cancer, that piece of tissue is a piece of a tumor, and scientists can use the resulting data to understand what genes are active within the tumor, what type of environment surrounds the tumor, and what macro-level processes the tumor is conducting. In theory, those scientists can use all of that information to determine the optimal treatment plan to destroy that tumor.
In order to understand scRNA-seq, you must first understand the concept of RNA — the molecule that functions as a copy of DNA that ribosomes use to build proteins. Continuing with our cooking analogy from previous posts, DNA is the master recipe that contains the instructions for building all of the proteins in your body; furthermore, as we learned last week, if DNA is damaged or altered, then the resulting proteins can become dysfunctional and create chaos throughout the cell — similar to how cooking a meal at the wrong temperature could result in catastrophe. For this reason, DNA resides in the nucleus in each of your cells, separate from the rest of the cell and protected by a highly regulated nuclear membrane. In order for a gene to be expressed — or turned on and translated into a protein — a signaling molecule must enter the nucleus and activate enzymes that copy the given gene, then that copy must be shipped back to the rest of the cell where ribosomes can use it to build the appropriate protein. RNA is the term used to describe the copy of DNA in that scenario, and the amount of a given gene’s RNA that is present in the cell depicts how much that gene is being expressed. In accordance with the analogy, imagine that the master recipe sheet (DNA) is locked away in a safe in the basement (nucleus) and cannot be transported to the kitchen (the rest of the cell), so you must copy down the recipe of interest (the gene), bring that copy (RNA) to the kitchen, and use that copy to build the meal (protein). Since different cells express different genes to different extents, scientists can distinguish different cell types — neurons, muscle fibers, red blood cells, etc. — from one another based on their RNA profiles.
In this study, the scientists did just that: they took samples from tumors, separated them down to single cells, tracked each cell with a labeled marker, extracted the RNA from each cell, and compared the RNA content of each cell to determine the different cell types and cell activities present in each tumor. Ultimately, they found incredible levels of variability between the different tumor samples, evidencing cancerous tumors’ abilities to adapt and specialize to their environments. “Our cellular analysis revealed remarkable heterogeneity for epithelial, immune and mesenchymal phenotypes existing within every tumor,” said the researchers, “We identified at least 9 major cell types that fall into 29 or 49 identifiable states at mid and high resolution, respectively. A number of these states most likely represent dynamic states along a continuum of differentiation, dependent on local interactions.” The researchers utilized Figure 1 below to depict the variability between tumors, as well as the diversity of cell types within each tumor.
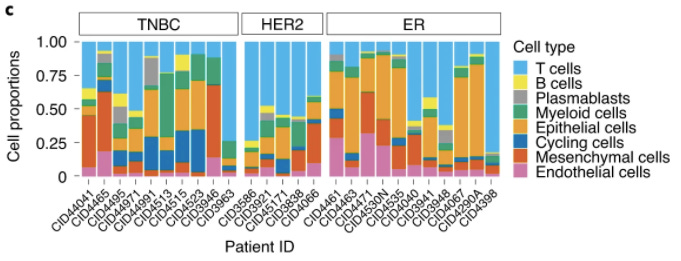
As you can see in the figure, no two tumors demonstrate the same cellular makeup, suggesting that each tumor exists in its own unique environment and posing a potential explanation for the variability between cancer patients’ responses to treatments. “While our study is not powered to make this inference,” said the researchers, “we hypothesize that intratumoral heterogeneity for intrinsic subtype may predict innate resistance to therapy and early relapse after therapy.”
Previously, physicians have utilized targeted chemotherapies, such as tamoxifen, to target specific receptors and pathways in certain vulnerable cancers; however, the data from this study suggests that determining those vulnerable cancers might require more than assigning tumors to clinical categories, as the tumors in each clinical category in the study demonstrated many differences in terms of their cellular makeup. In other words, a HER2 tumor, although distinguished by its clinical categorization and cell receptor profile, could be more similar in terms of cellular makeup to a given ER tumor than another ER tumor is. In the scope of tamoxifen and similar specialized drugs, this could mean that therapies that target a particular pathway or receptor might be extremely effective for certain tumors within a given macro category but minimally effective for other tumors within that same macro category.
Have you ever heard somebody propose a conspiracy theory that the government has a cure for cancer and is hiding it from us? Or maybe you have espoused similar language, questioning how we haven’t found a cure for cancer after we put billions of dollars into cancer research and treatment every year. If you don’t understand the fundamentals of cancer science, then these might be logical conclusions; however, it does not take much time in the world of cancer biology to recognize that the issue is far more complex than that: we need a cure for cancer, but each type of cancer is different, each patient with a given type of cancer has a unique tumor profile, each tumor within a given cancer patient has a unique cellular profile, and each cell within a given tumor has a unique genetic profile. So, unless we find a magic bullet that can eliminate ALL cancer cells without eliminating the all too similar healthy cells around them, then we will never have a universal cure to cancer as a category of diseases; instead, we must find a host of therapies and approaches to effectively destroy each patient’s specific cancer, all the way down to the genetic level.
In his book, The Emperor of All Maladies: A Biography of Cancer, Siddhartha Mukherjee said, “Down to their innate molecular core, cancer cells are hyperactive, survival-endowed, scrappy, fecund, inventive copies of ourselves...Cancer's life is a recapitulation of the body's life, its existence a pathological mirror of our own.” Much like us as early Homo Sapiens in the jungles of Africa, as Hunter-Gatherers in the forests of North America, and as intelligent beings in today’s world, cancer cells are evolutionarily driven, competing in Darwin’s game of survival of the fittest. This study exemplifies how cancer cells will follow a plethora of developmental tangents in order to surpass the restrictions within their environments, creating a blended rainbow of tumor diversity. If we are to defeat cancer, we must do what we’ve done time and time again to become the most dominant species on the planet: out-compete our adversaries. We must push our cognitive abilities to the max to develop better technology and more creative innovations, so we can adapt and overcome cancer.