Learning Lipids Part III: Explaining the Atherogenic Triad
"Perfectly balanced, as all things should be..." - Thanos, The Mad Titan
As an ROTC Cadet, infantry tactics act as the medium through which your leadership attributes and competencies are measured and assessed; however, you are not expected to be an expert infantryman, rather you are expected to think logically, critically, and effectively. For this reason, Cadets often find themselves faced with why-type questions: Why did you choose to place your security element here instead of there? Why did you only take three of your squad leaders on your leader’s recon? Why did you, as the platoon leader, choose to set the claymore yourself? Etc. If you’re high speed – meaning that you’re on top of your game – then you confidently defend your decision-making with sound lines of logic and/or doctrinal tactics. On the other hand, if you find yourself backed into a corner without clear reasoning at your disposal, you might bust out the classic cheat code: “It was/is METT-TC dependent.”
You see, in the world of ROTC, that 6-letter acronym serves as a, “get out of jail free,” card, in that you can whip it out as a catch-all when you don’t know how to explain your actions. In the world of health and fitness, you will encounter similar all-encompassing terms, like inflammation, insulin resistance, and toxicity, that people leverage as scapegoats to dodge complex questions that they don’t know the answers to. When pondering how to answer the questions I left you with in Part II of this Learning Lipids Series – Why do some people have larger lipoproteins than others, and what determines the concentrations of particles in your blood? – I found myself tempted to fall back on some grand, overarching theme as an easy escape. In the case of dyslipidemia, my scapegoat of choice is a classic one: metabolic dysfunction.
Though I will, in fact, lean on this vague and ubiquitous concept, I will at least define it in the context of our discussion below where we will take a step further into the lipidology rabbit hole, exploring how lipoproteins interact with one another, how energy balance can affect these interactions, and what this means for lipid biomarkers and CVD risk. Before we dive in, I encourage you to check out Parts I and II, along with my recap from last week below.
Medical Disclaimer: I am not a doctor, and none of the information below is medical advice in any way, shape, or form. Let this post serve as a spark to your curiosity and a stepping stone to conversations with your personal medical providers.
Recap
Cholesterol is a type of lipid, a compound that is nonpolar and does not dissolve in water as a consequence. In order to transport cholesterol and other lipids, your body packages them into protein complexes, called lipoproteins, composed of polar exteriors.
Lipidology can be simplified to the analogy of a highway, where the route represents a blood vessel, the vehicles represent lipoproteins, and the contents carried within/on the vehicles represent lipids.
Since the late 1950s, scientists and physicians have looked to cholesterol-based metrics to assess CVD risk; however, in recent years, expert cardiologists and lipidologists, like Allan Sniderman and Tom Dayspring, have challenged the status quo of CVD risk assessment, pointing to lipoprotein particle count as the best predictor of risk.
The low-density lipoprotein lineage is broken down based on density, with each particle’s protein-to-lipid ratio determining its density, in that the greater amount of lipids within the particle, the less dense the particle is and vice versa.
Very low-density lipoproteins (VLDLs) sit at the least dense end of the spectrum, as they contain the most amount of triglycerides and cholesterol. As those VLDLs offload lipids to cells throughout the body, they become denser and denser, until they are dense enough to be categorized as intermediate-density lipoproteins (IDLs). The process continues, as the IDLs ultimately relinquish their lipids, increase in density, and become low-density lipoproteins (LDLs).
When functioning properly, these VLDLs, IDLs, and LDLs make their way through your body – delivering essential lipids to muscle cells and endocrine glands in particular – and return to the liver, where they are removed from circulation and recycled; however, sometimes these particles find their way into the subendothelial space, or the area between the innermost layer of the blood vessel, the endothelium, and the next layer, the intima. If they become entrapped in this space, these particles can trigger an immune response that ultimately results in plaque formation, known as atherosclerosis.
Since the size and cholesterol concentration of LDL particles varies, multiple LDL particle concentrations can produce the same LDL cholesterol concentration.
For this reason, LDL-C can overpredict or underpredict LDL particle count, which can lead an individual to overestimate or underestimate their CVD risk respectively.
As you already know, lipoproteins serve as vessels for lipids, carrying cholesterol, triglycerides, and phospholipids throughout your body; additionally, you know that there are two main lipoprotein lineages: low-density (LDL) and high-density (HDL). We will likely dive in deeper on the latter family of lipoproteins in a future post, but, for the purposes of today’s conversation, you can think of HDLs as tow trucks in the scope of our highway analogy. When particles from the LDL lineage, also referred to as ApoB or Apo-B 100 particles, wander into the subendothelial space – analogous to a car going off of the road – they can become entrapped and oxidized.
This ultimately leads to an immune response, in which macrophages – easily envisioned as Pac-Man cells that eat up what they detect as foreign or harmful molecules – engulf the oxidized LDL particles and transition into foam cells when they accumulate a sufficient amount of cholesterol from those particles.
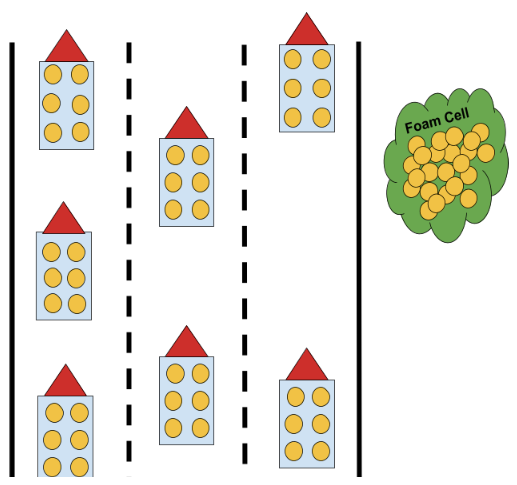
At this point, HDL particles are capable of traversing the subendothelial space and interacting with those foam cells to suck out their cholesterol contents and deliver said cholesterol to other parts of the body – similar to how a tow truck might pull a crashed car out of a ditch and bring it to a mechanic or junkyard.
Check out this article by Dr. Peter Attia for a deeper dive into the development of atherosclerosis.
This HDL functionality is the reason many view HDL activity as cardio-protective; though, as is exemplified by the many trials that have failed to improve CVD outcomes by raising HDL-C and as is emphasized by Peter Attia, Allan Sniderman, Ronald Krauss, and Tom Dayspring during their respective conversations on The Attia Drive, the HDL story is far more complex and multi-faceted than this brief picture portrays.
Now, as I alluded to earlier, Apo-B particles – mainly LDLs and VLDLs, since IDLs are cleared from the blood too rapidly – and HDL particles can interact with each other to swap their lipid contents. These interactions can be homotypic – meaning that two of the same type of lipoprotein exchange lipids – or they can be heterotypic – meaning that two different types of lipoproteins exchange lipids – and they are facilitated by cholesteryl ester transfer proteins (CETPs). So, what does this have to do with Apo-B particle size and concentration? In order to answer these questions, let’s retrograde back to that lazy and nonspecific term, metabolic dysfunction. In regards to dyslipidemia, you can think of metabolic dysfunction through the lens of energy balance, specifically the local energy balance of the liver. Your liver acts as a sort of control center, in that it processes all kinds of important molecules – like glucose, fatty acids, and cholesterol – and regulates their influxes, effluxes, and concentrations in your blood. Amongst these important molecules is the triglyceride – a lipid structure composed of three fatty acid chains bound to a glycerol backbone – which functions as a source of energy for your cells; consequently, the surplus, maintenance, or deficit of triglyceride can serve as a marker of energy balance in your liver and other cells throughout your body. In broad terms, when more, “energy,” is entering the liver than is being used or effluxed, a buildup of triglyceride develops, provoking your liver to increase triglyceride export in particularly triglyceride-rich VLDLs. This hepatic response ultimately sets off a chain reaction, the end product of which is a lipid profile infamously associated with increased CVD risk and commonly known as the atherogenic triad: hypertriglyceridemia, low HDL-C, and elevated small LDL particle count.
As is represented in Image A above, VLDL particles are relatively triglyceride-rich in comparison to LDLs and – though not depicted above – HDLs to begin with; consequently, the previously mentioned increase in triglyceride concentration widens this disproportion, prompting an increased lipid-exchange of VLDL triglyceride for HDL or LDL cholesterol.
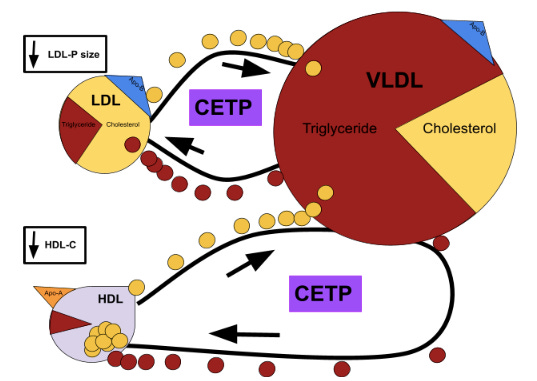
Check out this podcast with Tom Dayspring for a deeper dive into CETPs and other lipid-related topics.
In the case of the now cholesterol-depleted HDL particles, this increased exchange rate manifests as a low HDL-C; whereas, in the case of the LDL particles, the situation is slightly more complicated. The off-kilter energy balance that led to increased VLDL production and triglyceride-richness in the first place also causes an increase in hepatic LDL secretion. For this reason, LDL-C may remain moderate or elevated, despite the aforementioned increased exchanges with VLDL; however, once those triglyceride-rich LDL particles relinquish some of their triglycerides, their lack of cholesterol content can make them distinctly small. It is this combination of increased VLDL production, increased LDL production, and increased CETP exchange that materializes as an increased small LDL particle count, as well as an increased overall Apo-B count – something we described in Part II as a primary risk factor for CVD.
Here is where the story loops back to our discussion on discordance and the questions from above. If we return to the studies demonstrating discordance between cholesterol-based and particle-based metrics, we find that discordance is especially common in individuals with insulin resistance, Type-2 Diabetes, and Metabolic Syndrome, all of which correlate with metabolic dysfunction and elevated triglyceride. Although other factors play a role, such as unfavorable genetics related to Apo-B clearance, it appears that unhinged energy balance can alter lipoprotein size and concentration through increases in Apo-B particle production and particle-particle interactions, both of which increase the probability that one of those Apo-B particles swerves off of the highway into the subendothelial space, becomes oxidized, and propagates atherosclerosis.
References
#22 - Tom Dayspring, M.D., FACP, FNLA – part III of V: HDL, reverse cholesterol transport, CETP inhibitors, and Apolipoproteins. Peter Attia. (2022, May 6). Retrieved June 8, 2022, from https://peterattiamd.com/tomdayspring3/
Attia, Peter (2021, December 1). When does heart disease begin (and what this tells us about prevention)?. Retrieved June 8, 2022, from https://peterattiamd.com/heart-disease-begin-tell-us-prevention/
Jin, X., Yang, S., Lu, J., & Wu, M. (2022). Small, dense low-density lipoprotein-cholesterol and atherosclerosis: Relationship and therapeutic strategies. Frontiers in Cardiovascular Medicine, 8. https://doi.org/10.3389/fcvm.2021.804214
Lawler, P. R., Akinkuolie, A. O., Ridker, P. M., Sniderman, A. D., Buring, J. E., Glynn, R. J., Chasman, D. I., & Mora, S. (2017). Discordance between Circulating Atherogenic Cholesterol Mass and Lipoprotein Particle Concentration in Relation to Future Coronary Events in Women. Clinical chemistry, 63(4), 870–879. https://doi.org/10.1373/clinchem.2016.264515
Liou, L., & Kaptoge, S. (2020). Association of small, dense LDL-cholesterol concentration and lipoprotein particle characteristics with coronary heart disease: A systematic review and meta-analysis. PloS one, 15(11), e0241993. https://doi.org/10.1371/journal.pone.0241993
Mora, S., Buring, J. E., & Ridker, P. M. (2014). Discordance of low-density lipoprotein (LDL) cholesterol with alternative LDL-related measures and future coronary events. Circulation, 129(5), 553–561. https://doi.org/10.1161/CIRCULATIONAHA.113.005873
Pischon, T., Girman, C. J., Sacks, F. M., Rifai, N., Stampfer, M. J., & Rimm, E. B. (2005). Non–high-density lipoprotein cholesterol and apolipoprotein B in the prediction of coronary heart disease in men. Circulation, 112(22), 3375–3383. https://doi.org/10.1161/circulationaha.104.532499
Sniderman, A. D., Thanassoulis, G., Glavinovic, T., Navar, A. M., Pencina, M., Catapano, A., & Ference, B. A. (2019). Apolipoprotein B Particles and Cardiovascular Disease: A Narrative Review. JAMA cardiology, 4(12), 1287–1295. https://doi.org/10.1001/jamacardio.2019.3780
Xiao, Small, Dense Low-Density Lipoprotein-Cholesterol and Atherosclerosis: Relationship and Therapeutic Strategies: https://www.frontiersin.org/articles/10.3389/fcvm.2021.804214/full