Thus far in the Learning Lipids Series, we have covered some lipidology basics, such as lipid panel content and lipoprotein functionality, along with some nuances to the story, including the Apo-B Particle Model of Atherosclerosis, CETP activity, and the Atherogenic Triad. We’ve described elevated Apo-B particle metrics as primary CVD risk factors and painted off-kilter energy balance as one underlying culprit for producing those metrics; however, we have not yet explored the interventions available for combatting dyslipidemia. We’ve called out the, “villains,” of this tale, but we’ve yet to identify the, “heroes.” In the world of lipidology, you can split those heroes into two camps: pharmacological and lifestyle interventions. Here, we will outline some options from the former category, diving into mechanisms of action at both the systemic and cellular levels. Before we get started, I encourage you to refresh your memory by retrograding to my previous Learning Lipids posts (I, II, III) or reviewing the recap below.
Medical Disclaimer: I am not a doctor, and none of the information below is medical advice in any way, shape, or form. Let this post serve as a spark to your curiosity and a stepping stone to conversations with your personal medical providers.
Recap
Cholesterol is a type of lipid, a compound that is nonpolar and does not dissolve in water as a consequence. In order to transport cholesterol and other lipids, your body packages them into protein complexes, called lipoproteins, composed of polar exteriors.
Lipidology can be simplified to the analogy of a highway, where the route represents a blood vessel, the vehicles represent lipoproteins, and the contents carried within/on the vehicles represent lipids.
The LDL lineage is broken down based on density, and each particle’s protein-to-lipid ratio determines its density, in that the greater amount of lipids within the particle, the less dense the particle is and vice versa.
Very low-density lipoproteins (VLDLs) sit at the least dense end of the spectrum, as they contain the most amount of triglycerides and cholesterol. As those VLDLs offload lipids to cells throughout the body, they become denser and denser, until they are dense enough to be categorized as intermediate-density lipoproteins (IDLs). The process continues, as the IDLs ultimately relinquish their lipids, increase in density, and become low-density lipoproteins (LDLs).
Before we dive into the lipid-lowering drugs below, let’s build a framework around what we already understand to be correct about lipidology and CVD. As I mentioned above, if we take the position that the Apo-B Particle Model of Atherosclerosis is correct, then our zoomed-out view of intervention can start with the goal of lowering serum Apo-B particle count. From here, we can take two approaches:
1. Reduce the rate at which Apo-B particles are produced/secreted into the blood
2. Increase the rate at which Apo-B particles are cleared out of the blood.
With this in mind, our next move is to target the, “players,” associated with those two processes. For Apo-B production/secretion, this means focusing on mechanisms associated with synthesis and storage; whereas, for clearance, this means focusing on hepatic receptors responsible for pulling Apo-B particles out of the blood. In the world of lipidology, there are a handful of approaches designed to attack these types of mechanisms – many of which ultimately attack both production/secretion and clearance – and, now that we have established a framework to view them through, I’ll use a few famous options below to highlight some of the different lanes available (pun intended).
Statins
If you know anything about cholesterol medications, chances are you’ve heard about statins, the king of them all. Since the late 1980’s, statins have served as the most popular and arguably most effective group of drugs in regard to treating hyperlipidemia. At this point, 7 statins – rosuvastatin(Crestor), atorvastatin(Lipitor), pitivastatin(Livalo), pravastatin(Pravachol), simvastatin(Zocor), lovastatin(Altoprev), and fluvastatin(Lescol) – sit on the market, each of which contains its own side-effect and efficacy profile, but all of which act through the same central mechanism: HMG-CoA reductase inhibition.
HMG-CoA reductase serves as the second of nine enzymes involved in the 37-step process of cholesterol synthesis – also known as the mevalonate pathway – during which a molecule of acetate transforms into the ultimate 4-ring structure. Statins act as reversible competitive inhibitors of HMG-CoA reductase and road blocks to cholesterol synthesis as a result. This action impacts Apo-B count in two ways, the first of which is intuitive: it reduces the amount of Apo-B particles the liver constructs and secretes. In addition, by depleting the liver’s cholesterol pool, statins provoke an upregulation in the expression of LDL-receptors (LDLR) – the proteins that suck Apo-B particles out of the blood and into the liver – to replenish that depleted cholesterol pool. This second mechanism is the main lever by which statins decrease serum Apo-B concentration. Statins typically produce relative reductions of LDL-C by 20-50%, Apo-B count by 10-30%, and 5-year CVD risk by more than 20%, which explains their place at the top of the CVD pharmacology food chain.
PCSK9 Inhibitors
In 2015, PCSK9 inhibitors (PCSK9i) took to the streets of clinical lipidology, offering a novel mechanism of reducing Apo-B through increased hepatic LDLR concentration. This time, instead of depleting the hepatic cholesterol pool, the aim is to target the LDLR cycle directly by preventing proprotein convertase subtilisin/kexin type 9 (PCSK9) enzymes from degrading LDLRs. Ordinarily, hepatic LDLRs bind with Apo-B particles, import them into the liver, drop them off in lysosomes for degradation and recycling, and return to the cell membrane to repeat the process; however, the LDLRs have a life cycle consisting of production, functioning, and degradation too, in which PCSK9s facilitate the lysosomal degradation step – a taste of the LDLRs own medicine. By inhibiting PCSK9s, we can increase the lifespan of each LDLR and increase the hepatic LDLR concentration as a consequence; furthermore, as we already established, in theory, doing so will reduce serum Apo-B concentration and CVD risk.
And, for practical purposes, this is exactly what the data suggests, showing that PCSK9 inhibitors lead to relative reductions of LDL-C by ~45-60%, Apo-B by ~20-40%, and major acute coronary events (MACE) by ~15% after ~2 years when given to patients already taking statins. The first generation of PCSK9 inhibitors – evolocumab(Repatha) and arilocumab(Praluent) – utilize PCSK9 antibodies to achieve their inhibition; whereas, the second generation – inclisiran(Leqvio) – leverage small interfering RNA (siRNA) to disrupt PCSK9 expression within the liver. Interestingly, inclisiran treatment consists of a baseline injection followed by a second injection 90 days later, after which patients receive injections once every 6 months. For reasons surrounding consistency and ease of use, this therapy may substantially alter the face of dyslipidemia treatment.
Cool Bonus: In patients with familial hypercholesterolemia(FH), a genetic condition that induces elevated LDL metrics, individual responses to different classes of lipid-lowering therapies can vary based on mutation variability. For example, patients with homozygous LDLR-negative mutations do not respond to PCSK9 inhibitors as well as patients with different FH mutation profiles.
Ezetimibe
At this point, you might be wondering what pharmacological interventions we have for countering dietary cholesterol consumption because, after all, doesn’t the cholesterol we eat dictate the levels of cholesterol in our blood? This thought is intuitive, especially considering the – in my humble opinion – misinformation thrown around online and in the media regarding dietary cholesterol; however, most of the cholesterol we consume we cannot absorb due to chemical incompatibility. In short, cholesterol comes in two flavors: unesterified and esterified. The latter carries a bulky ester group where the former carries a hydroxyl group, and the Niemann-Pick C1-like proteins that line your enterocytes (the cells of your small intestine) utilize this structural distinction to filter out the cholesterol molecules with that bulky side chain. With this in mind, and since much of the cholesterol we eat is esterified, it makes sense that dietary cholesterol does not heavily impact serum cholesterol – unless there exists dysfunction in the selective enzymes involved in the absorption process. That said, we do absorb unesterified cholesterol from our gut, but the majority of it comes from the bile acids our livers excrete.
Now, some individuals, commonly known as hyperabsorbers, exhibit a faulty filter in terms of cholesterol absorption and uptake too much cholesterol; furthermore, some individuals experience an increase in intestinal cholesterol absorption as a homeostatic reaction to lipid-lowering therapy. In both cases, ezetimibe(Zetia), a NPC1L1 inhibitor, can combat dyslipidemia by reducing intestinal cholesterol absorption and lowering Apo-B concentration as a result. In addition to intestinal absorption, ezetimibe can also impact biliary absorption, in which the liver imports cholesterol from bile acids it previously secreted into the hepatic ducts. Given in conjunction with a statin, ezetimibe offers additional relative reductions of LDL-C by ~15-25%, Apo-B by ~10-15%, and MACE by ~7%.
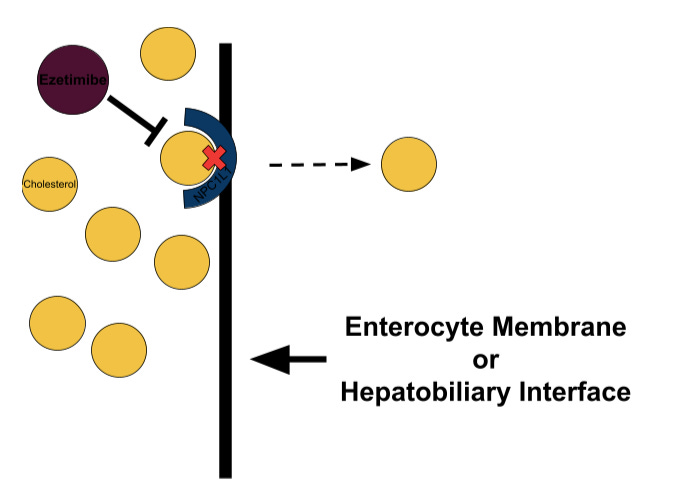